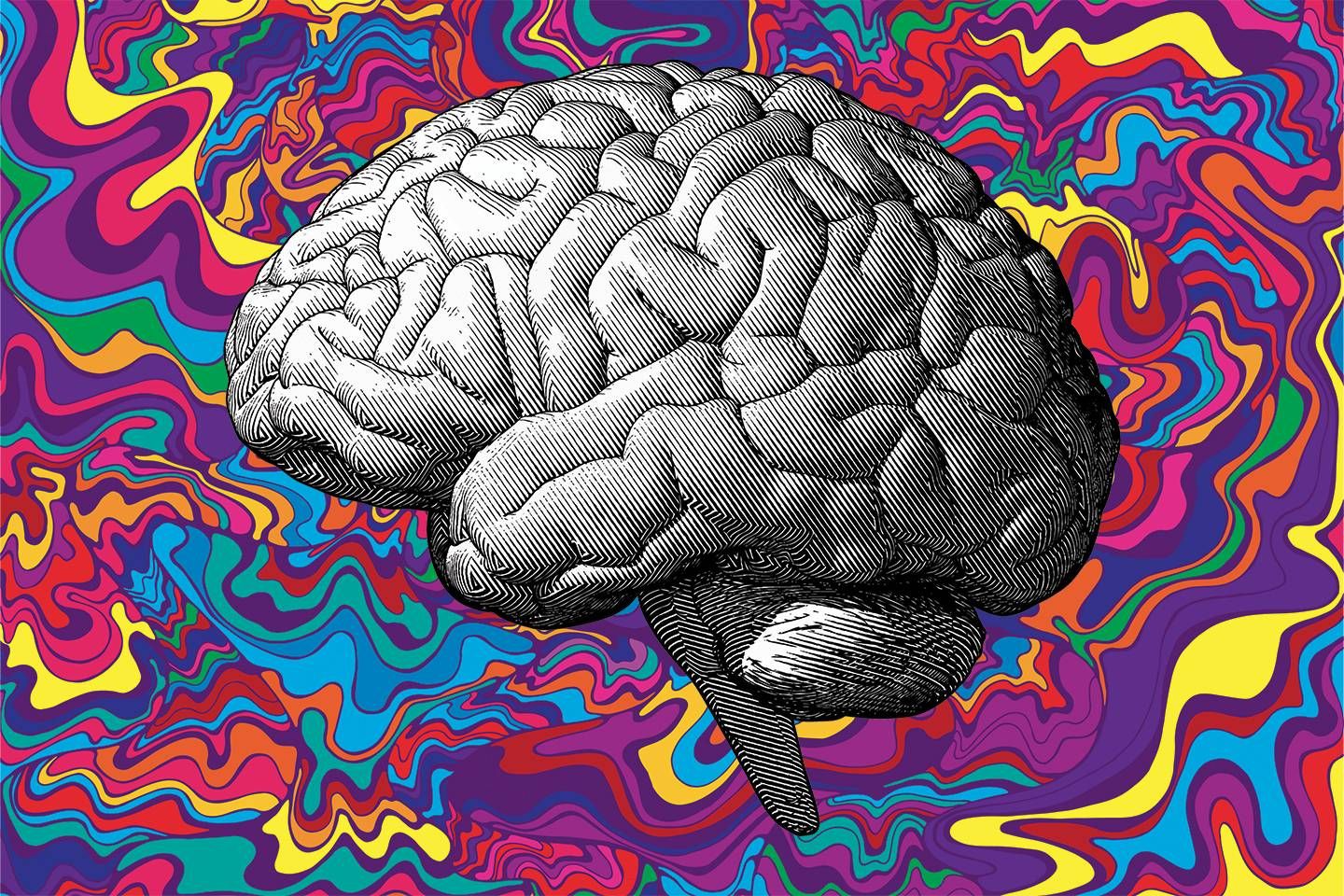
Here’s What Neuroimaging Can Tell Us About the Psychedelic Experience
Image via
There’s no doubting your status as a psychonaut. You’ve most likely tripped on a variety of substances stored in small plastic baggies shoved in the backs of drawers or locked away inside decorative wooden boxes. Your aunt (the cool one) now sends you sporadic articles of how the same psychedelics you used to talk about self-transforming machine elves with are now the subject of numerous studies to treat an array of cognitive disorders like depression, PTSD, alcoholism, and severe drug addiction, to name a few. None of this is surprising to you considering how you’ve changed as a person since taking psychedelics. However, you’re now at the point in life where you want to dive just a bit a deeper into the psychedelic experience. Specifically, you want to know the science behind it all.
Well, you’re in luck. Consider this an introductory crash course in the realm of neuroscience and psychedelic research. You won’t get overwhelmed, in fact you’ll digest complex neuroimaging techniques with ease. Neuroscience is to the brain what astrophysics is to the universe. Of course, combine neuroscience with a tab of LSD and things get really interesting.
We’re first going to go over the variety of neuroimaging techniques that peer deep into the brain. Then, we’re going to dive down the vortex of the psychedelic experience and explain why your brain does what it does when you trip on a substance. Next, we’re going to go over a handful of peer-reviewed research studies surrounding some of your favorite psychedelics — and by the end, you’ll want to stop what you’re doing and become a neuroscientist to lead the psychedelic research revolution. At the very least, you’ll be able to respond to your aunt with something more than, “Yeah, that’s really dope.”
The Science of Neuroimaging
Historically there are a handful of ways to measure brain activity through neuroimaging. If you’ve watched any contact sport you’ve likely heard of CT scans, a common imaging process professional athletes undergo to detect injuries and problems with blood flow. Computed tomography (CT) scans use blasts of radiation to construct a map of the brain. The stronger the radiation, the clearer the image — which is concerning considering that each scan can be up to 500-times (or higher) the radiation of a standard x-ray.
Another neuroimaging technique, Positron Emission Tomography (PET), uses injections of radioisotopes (or, atoms that have increased nuclear energy) and tracks the path of these particles to form a blueprint of a person’s brain, including any abnormalities. Still, with PET, radiation plays a primary factor in neural imaging. While nuclear medicine can be helpful when science can’t effectively map neurophysiology without radiopharmaceuticals, it’s best not to blast anyone — or anything — with unnecessary amounts of radioactive material, especially if more effective technology exists. That is, unless you’re living inside of a comic book universe — then use all the gamma radiation you want. You might create the next Avenger.
This leaves three remaining neuroimaging techniques: One of which is the preferred method in psychedelic research; the other a bit antiquated, but still may serve some purpose; and a third that’s so expensive we don’t even know its full potential in psychedelic science because it’s that luxurious.
At nearly 100 years old, electroencephalography (EEG) is undoubtedly the elder of the neuroimaging squad and by far the most affordable. EEG works by detecting electrical currents from the brain through the use of electrodes affixed inside of a skullcap that a wearer uses. It’s not the most fashionable item, but EEG has high temporal capability, which means it’s able to detect neurological changes almost immediately. Each electrode inside an EEG cap can detect the electrical currents from 30 to 500 million neurons. That’s per each electrode — some of the more sophisticated EEG systems can comprise of 256 electrodes attached to a skullcap with a capacity to detect the electrical waveforms of up to 128 billion neurons. You didn’t even know you had that many neurons rhythmically firing off, but you do — and you just used a lot of them to read this sentence.
There are a multitude of applications for electroencephalography. Due to it’s high temporal value, EEG shines at detecting epilepsy, sleep disorders, dementia and any other structural brain disease or disorder. Now that EEG technology is getting cheaper (you can actually grab a single-electrode unit for about $100), some are using it to track their brain activity when they’re gaming, learning, and just to keep a track of their daily grind. Keep in mind, you basically get what you pay for in neuroimaging. If you want a more complete and detailed electro-mapping of a brain, high-end EEG devices can reach up to $124,000.
Of course, if you want to dive into the quantum-mechanical monitoring of your brain activity with the most temporally precise neuroimaging device ever created — it’ll cost you a cool $2 million.
Magnetoencephalography (MEG), the beginning pronounced exactly like the X-Men super villain “Magneto” and the rest you can just hear me pronounce. While EEG measures electrical currents from your brain, MEG measures the electromagnetic field oscillation of the electrons produced by your dendrites during synaptic transmission. It can also detect the activity of as few as 50,000 neurons (compared to the millions from EEG), which leads to an incredibly accurate temporal image of the brain.
The way MEG works is Maxwellian in its complex ingenuity. It’s also quantum science considering every magnetoencephalography is equipped with a superconducting quantum interference device (SQUID). There’s a large science vortex that we can fall into at this point, and it’s filled with mathematical equations that look like extraterrestrial hieroglyphics. What you need to know is since MEG measures the electromagnetic field fluctuations of neurons, it’s a hyper-sensitive device that has incredible temporal accuracy in the range of milliseconds. This means scientists can witness the precise sequence of cognitive events as they occur in the brain instead of a nebulous cloud of brain activity. The sensitivity of MEG is so powerful that it needs to be shielded from the Earth’s electromagnetism because it will affect the measurement of the device. Oh, and it’s massive. It looks like a hairdryer for giants.
While the temporal quality of both EEG and MEG are remarkable, psychedelic research isn’t so much concerned about when a person’s trip begins and ends as it is about which parts of the brain are actually activating — the spatial accuracy. That’s not to say EEG and MEG don’t serve a purpose within psychedelic research. Applying neuroscience to psychedelics is still a fairly new field — one of the earliest known neuroimaging studies into psychedelics is a scientific paper titled, “Neuropsychiatric manifestations following the use of 3, 4-methylenedioxymethamphetamine (MDMA; ‘Ecstasy’),” co-authored by Richard S. Cohen and James Cocores in 1997. The paper is pretty ominous in its portrayal of MDMA, but the researchers recognized the limitations of temporal-based EEG and sought a new way to spatially research the brain through an earlier technique called Brain Electrical Activity Mapping (BEAM) (now called QEEG), the spatial precursor to Magnetic Resonance Imaging (MRI) and later Functional Magnetic Resonance Imaging (fMRI), the most promising neuroimaging technique for psychedelic research.
So why is fMRI such a sought-after neuroimaging technique in psychedelic research? Well for starters, it’s not the price of a private island nor does it involve potentially harmful nuclear energy. The key to fMRI’s success in researching psychedelics is its incredible spatial ability — an opportunity to see what parts of the brain are activated while tripping on psychedelics.
fMRI has a temporal accuracy of seconds and a spatial accuracy of millimeters, and since it hit the scene in 1991 it has steadily developed to be the primary choice for non-invasive brain imaging. The technology of fMRI’s brain imaging is BOLD (Blood oxygen level-dependent). When parts of your brain are active, it requires an increase in oxygen which, in turn, requires more blood to flow in the area. The blood that your brain receives during these times of activation is oxygenated blood, or ‘oxyhemoglobin.’ When a part of your brain becomes active, the non-oxygenated blood quickly evacuates the scene, within seconds actually. fMRI is genius in that oxygenated blood has a different magnetic resonance signal than non-oxygenated blood, and fMRI has the capability to detect the difference and creates a spatially accurate map of the brain. With fMRI we can identify which regions of the brain are active, have functional connectivity (when two or more parts of the brain simultaneously are active) in order to build a visual map of cognitive processes occurring. It also yields to beautiful fMRI images like this which are inherently pretty friggin’ psychedelic
The brilliance of fMRI is that it represents innovations in physics, engineering, chemistry — science teamed together and created a literal brain-reading machine — and now we’re going to use it to determine just how hard a person trips on psychedelics and what exactly happens to the brain when a person is effortlessly flopping in between trippy dimensions.
The Science of the Psychedelic Experience
If you’re this far in the article, there’s a strong possibility that you’ve already tripped and are genuinely interested in learning how neuroimaging can be utilized to better understand the psychedelic experience. There’s even a chance that you’re tripping right now.
All psychedelics loosely belong to two categories: serotonergic psychedelics, or most tryptamines, and dopaminergic psychedelics, which consists almost entirely of phenethylamines. This isn’t like the indica and sativa of cannabis (which actually doesn’t even exist). Tryptamines are psychedelics that bind to serotonin (5-HT) receptors by being what’s called an agonist, or a substance that initiates a physiological response when combined with a receptor. Dopaminergic psychedelics operate on the same principle, except they use the dopamine neurotransmitter to deliver your trip. Even with dopaminergic psychedelics we still find evidence these also bind with serotonin receptors, along with other receptors.
The role psychedelics operate on these neurotransmitters is largely not understood. We still don’t fully know why we trip, and that may bother some people — but it’s the reality that we all inhabit. However, the emerging consensus is that humans experience psychedelic trips due to an interruption in what’s called the Default Mode Network (DMN) — and if you’ve never heard of the DMN, its existence within the brain is as trippy as any psychedelic you could imagine.
To set a control for a neuroimaging experiment, participants are told to relax and close their eyes while a device like fMRI captures their brain’s resting potential. The resulting neuroimage was considered ‘noise’ and when the real brain activity from a cognitive task happens within the experiment, the noise was subtracted from the finalized neuroimage so that researchers can identify which parts of the brain were active. This technique remained the dominant perspective within neuroscience until 2001 by a stroke of genius from neurologist Marcus Raichle.
There’s a lot we can say about Dr. Raichle — like when he climbed an 18,000 ft mountain in Pakistan just to inject himself with radioactive xenon. At a time when the entire field of neuroscience was subtracting the brain’s resting-state noise from an experimental cognitive task, he did the opposite and subtracted the entire cognitive task from the noise in order to see which parts of the brain were essentially ‘turned off.’ What he found is the brain is continuously busy in a state of low-frequency oscillations, like when you have apps running in the background of your phone. When a cognitive task is present, your brain has to turn off certain things it’s already doing in order to attend to the external stimuli. What was considered ‘noise’ was now identified in 2001 by Raichle as the Default Mode Network.
With his discovery, he crushed the theory that higher functional brain activity only exists when a cognitive task is present. In just a few years after his discovery, we now know that 90% of the energy consumed by the brain is used to support the DMN — and it’s active during sleep and light anesthesia. The DMN spans several cortical areas of the brain and is responsible for an array of unconscious activities and semi-unconscious but deeply cognitive things like daydreaming, imagining the future, and introspection. The DMN is now an established scientific fact and suggests that perhaps the concept of life shouldn’t wholly be focused on the conscious as much as it should be on the unconscious.
When the DMN is interrupted or suppressed by psychedelics, the ‘trip’ starts to happen — and it could lead to some interesting cognitive events that have practical value in society. University of California’s Jennifer Mitchell’s groundbreaking work on MDMA unraveled its extraordinary ability to treat people suffering from PTSD. Psilocybin and LSD have shown a remarkable potential to help treatment-resistant depression. Results from a clinical potpourri of neuroimaging studies have unveiled the potential psychedelics have on improving functional activity in cognitive disorders by utilizing neural networks in the DMN.
However remarkable those breakthroughs may seem, the fact remains that humanity just doesn’t know enough about neurology (especially molecular neurology), let alone the neurological effects of psychedelics. It’s not due to a lack of trying. Psychedelic research hasn’t been the primary driver for government-issued grants or university-funded projects. We’re trying to navigate a substance that’s federally illegal, and the only trail of objective evidence we have is mostly generational lore, lots of survey-based research, and morsels of neuroscientific analysis and research in specific countries in which their governments and educational institutions permit psychedelic investigations.
It’s also important to note that there may be vastly more psychedelics that are yet to be discovered, including substances that bind to other neurotransmitting sites in the brain beyond serotonin and dopamine. Undiscovered psychedelics (both natural and synthetic) that could be cholinergic (which uses the parasympathetic nervous symptom, responsible for sexual activity), adrenergic (relates to adrenaline), and more sophisticated racetams (not the useless ‘neurotrophic’ drinks you see in corner stores) that assist with cognitive performance and memory retention. Those are just some. The evolution of neuroscience over time may lead to the discovery of new psychedelics binding on to neurotransmitters that we don’t even know about yet.
What Neuroimaging Tell Us About the Psychedelic Experience
So which significant neuroscientific psychedelic studies have already been conducted? Implementing neuroimaging for the purpose of investigating psychedelics is still a fairly new concept, and you can even see it in the scientific literature. We’ve gone from hallucinogens to psychedelics, from ‘drug abuse’ to ‘drug use.’ Even with all the impediments in the advancement of neuro-psychedelic research, there’s an ever-increasing body of scientific work that’s being assembled across the world. I’m going to focus on three of the most popular psychedelics (MDMA, psilocybin, and LSD) and go through a few peer-reviewed research articles on how fMRI was used to reveal our neurological relationship with these substances. Keep in mind there are many more, and I encourage you to dive in a vortex of late-night psychedelic research. However, at this point, I’m sure you have better things to do, like dusting off the bag of shrooms your friend gave you that you’ve been contemplating taking since the beginning of the pandemic.
MDMA
A lot of people flip out when they realize their favorite festival psychedelic methylenedioxymethamphetamine actually has ‘methamphetamine’ in the name. Just because the name includes the name ‘meth’ doesn’t actually make MDMA meth. That’s like thinking volleyball and basketball are the same sport because they share a round ball.
The research on MDMA is setting its own stride in the neuroscience community and some incredible correlations are being discovered through fMRI. Here are just a few interesting research studies.
(Feduccia 2008):
Ph.D. researcher Allison A. Feduccia wanted to test the impact music and MDMA had on the brain. The University of Texas wouldn’t allow her to use human participants for the study, so she recruited 100 laboratory mice, gave them an injection of MDMA, and bumped ‘The Very Best Euphoric House Breakdown’ in their cages. What she discovered is that the rewarding factor of MDMA was dependent on the music (EDM or white noise) and dependent on the ‘set,’ or environment of the rats (whether they were in a separate cage or out in a communal space). Listening to music and processing the rhythm makes the mesolimbic dopaminergic system active, which means good music increases dopaminergic neurotransmission. Feduccia’s work suggests that since MDMA and music share similar neural pathways, the combination of both influences each other in a positive way. Probably explains why taking MDMA and going to festivals is so damn enjoyable.
(Daumann et al. 2003):
In Jörg Daumann’s research that was published in the journal Cognitive Brain Research, he wanted to see if there were any long-term memory impairments that resulted from people that have a history of taking MDMA. He found that heavy MDMA users had a weaker blood oxygenation level-dependent (BOLD) response in their fMRI scan, but there were no significant long-term memory differences between people that have a history of taking MDMA and people that have never used it. There is no scientific evidence MDMA has a negative effect on long-term memory.
(Valdes et al. 2006):
Another fMRI-enabled psychedelic study investigated the idea that MDMA users may be more impulsive than people that haven’t consumed the substance. Aside from using neuroimaging, researchers implemented the Barratt Impulsiveness Scale (BIS) test which served as another way to gauge the impulsiveness of the participants. fMRI scans showed MDMA users had greater activation in their prefrontal cortex, an area of the brain known to control impulse. The result of the study concluded that MDMA users were more impulsive than non-MDMA users. This could also mean that people with generally greater prefrontal cortex activity have a neurological predisposition to take MDMA and other psychedelics.
(Carhart-Harris 2015):
In 2015, Robin L. Carhart-Harris (now the head of the Centre for Psychedelic Research for Imperial College London) conducted an fMRI study in which 25 participants dosed on MDMA and mapped the cerebral blood flow and resting-state functional connectivity. The results reinforced that blood flow is reduced in certain parts of the brain after administering MDMA — the reduction of the blood flow is congruent with the subjective intensity of the trip. Essentially the higher the cerebral blood reduction in the right amygdala and hippocampus, the greater the trip. Robin also noted that people that took MDMA were generally in a more positive mood, a phenomena that most likely surprises no one.
LSD
Lysergic Acid Diethylamide, the only psychedelic that has its own holiday. Coincidentally LSD and the existence of serotonin were discovered right around the same time in the 1950s, and out of all the psychedelics mentioned in this article, LSD is undoubtedly the most potent. In fact it’s so potent that it’s the only psychedelic that has a measured dosage of microgram (μg) instead of milligrams.
The fMRI research on LSD is some of the most abundant, but here are just a few studies that begin to unravel the substance’s innate mystery.
(Bershad 2020):
RZA is the Anya K. Bershad of hip-hop — which is perhaps the greatest compliment I’ve ever bestowed on any living human being. When it comes to her rapidly emerging work in serotonergic drug research, she’s one of the most referenced psychedelic scientists this year, and for good reason. Her work investigates topics that are virtually unheard of, like how MDMA changes socio-political views.
In her study published in the April 2020 issue of Biological Psychiatry: Cognitive Neuroscience and Neuroimaging, Bershad wanted to investigate the recent trend of microdosing LSD and analyze if there’s any scientific merit to the practice. She gave 25 participants 13 micrograms of LSD (a standard amount for a microdose) or a placebo in a double-blind experiment. Bershad then used fMRI to see resting-state functional connectivity cerebral blood flow, which was also followed up by a questionnaire to assess mood. The results showed that microdosing altered some connections within the amygdala, but overall it had little to no effect on the mood of the participants — a common result that we’ve seen in regards to studies about microdosing.
(Kaelen et al. 2016):
As if you needed more reason to trip and listen to good music; Mendel Kaelen’s study on LSD modulating music within the brain is pretty intriguing. There’s a part of the brain called the parahippocampal cortex that has been linked to music-invoked emotion, mental imagery, and is also an active site while under psychedelics. When participants were dosed with LSD and listened to music (the album Yearning by ambient artist Robert Rich and composer Lisa Moskow, since you just had to know) with their eyes closed. What Kaelen saw was an increase in functional connectivity between the parahippocampal cortex and surprisingly the visual cortex. This indicates that LSD and music activate the visual cortex in an interesting way, especially with a lack of visual stimuli.
(Sanz et al. 2021):
For a brief period of time, psychedelics were called ‘psychotomimetic’ because they mimicked a state of psychosis within the user. An indicator of a state of psychosis is disorganized speech, a premise that Argentinian researcher Camila Sanz ran with in her recently-published work in the journal of Consciousness and Cognition. She used fMRI to scan the brains of 20 participants that were either injected with LSD or a placebo. They were then interviewed at the peak of their LSD trip, and Sanz discovered the verbosity of words increased while the lexicon of what was said decreased. Basically, they were saying a lot that meant very little. The interviews were transcribed and machine-learning was used to further analyze words and grammar. Camila Sanz concluded the phenomenon of disorganized speech was a landmark feature of tripping on LSD.
Psilocybin
One of the active ingredients in magic mushrooms, psilocybin (the other ingredient is psilocin), has the least amount of peer-reviewed scientific research than the previously mentioned psychedelics. I’m not sure why that is, considering shrooms are by far the most accessible. Any person with a container the size of a shoebox can start their mycelial empire if the initial growing conditions are up to par. There are many mushroom cultivation resources that provide a full framework for anyone that wants to give it a shot — but, this article isn’t it. We’re focused on the science, specifically the science behind psilocybin. Check out these select fMRI psychedelic studies to get a clearer understanding of your favorite fungus and the only brain you know.
(Barrett 2020):
There’s an area of the brain called the claustrum and it’s an active site for serotonin receptors. It also provides glutamatergic inputs (energy) to arguably all areas of the brain. Researchers suspected psilocybin has the ability to alter how the claustrum functions, so they set up an elaborate study where participants’ brains were scanned 100 minutes into chowing down on shrooms. One of the most notable things they discovered was psilocybin significantly decreased functional connectivity of the right claustrum with auditory systems and the default mode network. At the same time, psilocybin increases connectivity of the right claustrum with the frontoparietal task control network — a system critical for controlling goal-driven behavior. There’s a lot to take from the study, and it only raises more questions than it does answers. However it’s the first study in history to look at serotonin receptors and psilocybin influencing the claustrum, and it just happened last year.
(Roseman 2018):
Another from the Imperial College of London; people with treatment-resistant depression were given psilocybin, shown faces that were neutral, fearful, and happy, then their amgyldas were fMRI scanned. Each participant was also provided psychological support during the study. The researchers expected that response to these faces (especially the negative and neutral ones) would improve after psilocybin and therapy, giving evidence that both practices improve treatment-resistant depression.
What they found was exactly that, shrooms mixed with psychological support rapidly improves depression. In fact, psilocybin increased emotional stimuli in each individual, something Leor Roseman and his colleagues didn’t expect. Once again, we have empirical evidence that another psychedelic can serve a critical role in treating depression with people that are generally incapable of alleviating their depression.
(Carhart-Harris et al.2012):
The reason we know as much as we do about psilocybin’s effect on the brain comes directly from this 2012 study that combined the efforts of six institutions across Europe. It started like any groundbreaking study should, gather up a bunch of healthy individuals and dose them with psilocybin. fMRI imaging was conducted and researchers discovered that psilocybin decreased cerebral blood flow in several areas of the brain, mostly in the thalamus and the medial prefrontal cortex. What’s interesting about this finding is the decreased cerebral blood flow was directly correlated to the intensity of the psilocybin trip, the first time this phenomenon has ever been recorded to this magnitude. Researchers believed the unexpected mind alterations from psychedelics enabled ‘a state of unconstrained cognition.’
Conclusion
Look at you and your newfound knowledge of neuroscience and psychedelics. Just take some time to congratulate yourself. If you can get through this extensive article on the science of the psychedelic experience filled with bad comic references, then you can literally do anything. The truth is, the field of neuroscientific psychedelic research is so new that we need people like you to enter it. It doesn’t matter what age you are or where on the planet you call home. Unraveling the mysteries of the brain is open reign for anyone that wants to do it, including yourself. There are a wealth of online resources you can tap into to further your knowledge and when it comes to higher education, universities across the world are opening their institutions to neuroscience and psychedelic research. For all I know, the next research study I quote may be from you — or your aunt. She’s obviously into this stuff too.
Post a comment: